From Flower Design to Designer Crops
Plants have been a long-time constant in Andrea’s life.
As a high school student, she was fascinated in every moment of her biology class. After school, she was beginning her side gig as a floral designer, which she continued throughout college and beyond. “I always appreciated the variety in shapes, sizes, and colors of the flowers. I wondered why they looked so different.”
Then, as a freshman at SUNY Binghamton, Andrea took a botany class and was hooked. She pursued biology as an undergrad and upon graduation was awarded the John D. Grierson foundation award for excellence in botany. After that, her creative spirit took her to the west coast to continue floral design and interior plant-scaping in San Diego. She certainly ended up there for a reason: Still curious, she applied for a position as a lab assistant at Torrey Mesa Research Institute (TMRI), which was a decision that would change her life forever.
“It was an epicenter for plant molecular biology and it was all so new. Everyone was so excited – you could just feel the energy,” explains Andrea. Molecular biology was new to Andrea, but the excitement was infectious, and she had to learn more. She decided to go to graduate school for a PhD in plant molecular biology. Little did she know the best was yet to come. “And then I learned about developmental biology,” she says. “It was a game changer for me.” Now there was a scientific basis for why those flowers looked different.
The Architecture of a Plant
Andrea’s team now uses developmental biology combined with new approaches in data science and phenotyping to understand the genetic and molecular basis for plant form. The architecture of a plant, such as the position and arrangement of branches, leaves and flowers, has major implications on crop yield.
“A plant’s shape is a key factor in determining its productivity. If we can understand the mechanisms that control plant architecture, we can improve crops to yield more, more sustainably,” explains Andrea. “For example, leaf angle and orientation in a field can be optimized for increased planting density while at the same time maximizing light capture for photosynthesis.” To understand plant architecture, Andrea and her lab study the genes and gene networks that control the development of plant organs from meristems, which are pools of plant stem cells.
Her lab also studies the impact of new environmental challenges on plant shape. “We want to understand how the molecular mechanisms that control plant shape are influenced by extreme environmental stresses, such as water- and nutrient-limiting conditions,” says Andrea. “We can also learn something from plants that are more adapted to these stressful environments.”
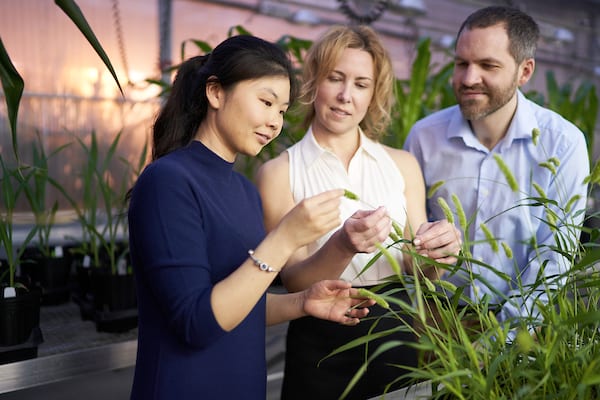
Sorghum and Sustainable Bioenergy
One crop that Andrea’s lab studies extensively is sorghum, which has natural resilience to drought and heat stress and can thrive on minimal nutrient inputs. Andrea’s lab explores the gene networks underlying this amazing stress resilience so they can define genetic elements that confer tolerance. To understand the complexities of drought response in the broader context of the whole plant and its environment, Andrea and her lab use advanced approaches in genetics, genomics, phenotyping and gene editing. Eventually, her work with sorghum could help improve the crop to be used as a sustainable bioenergy feedstock for fuel production.
“In the coming years it is inevitable that globally we will need to produce more food and alternate sources of fuel more sustainably. I think we can do that with predictive science and crop improvement through technology,” says Andrea.
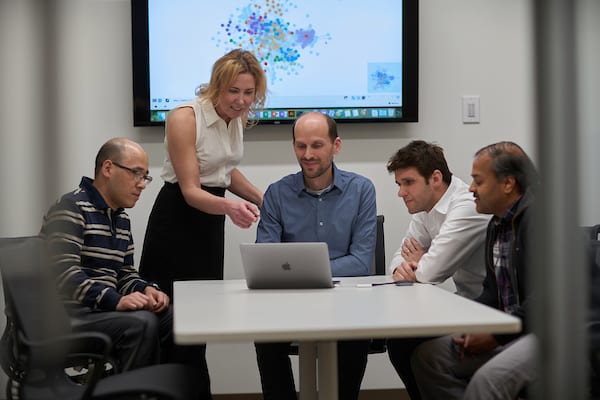
The Next Question
Today, Andrea still harnesses the creativity that first drew her to floral design. “People might not realize it, but biology is a creative science. The more creative we can get about integrating data that we collect and how we visualize and share it, the clearer new insights become,” she says.
Andrea and her lab are always thinking of the next question to ask so they can continue to make a bigger impact. “My group is enthusiastic about the work they are doing. It is an exciting time to be in plant biology,” Similar to her experience at TMRI twenty years earlier when the first plant genomes and microarrays were coming available, advances in phenotyping and genome editing are now enabling a new level of opportunity for crop improvement. “I can clearly see the potential impact and it makes my work exciting and meaningful,” explains Andrea.
And she’s just getting started. “There is still so much that we don’t know. For each insight that you have, ten new questions arise. Often there are many ways to get to a common answer. Biology is extraordinary like that!”
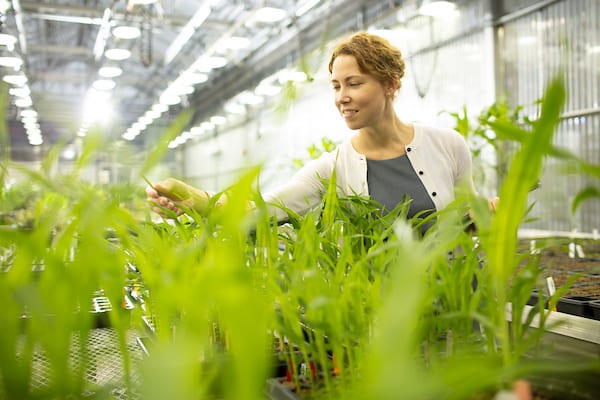